Dr. Qing Wang | Naval Postgraduate School
Presenting: "Quantifying the Impact of the Atmospheric Boundary Layer on Optical Signal Propagation"
Hosted by the Physical Oceanography Lab
MLML Seminar | April 9th, 2025 at 4pm (PDT)
Watch the Live Stream here or here
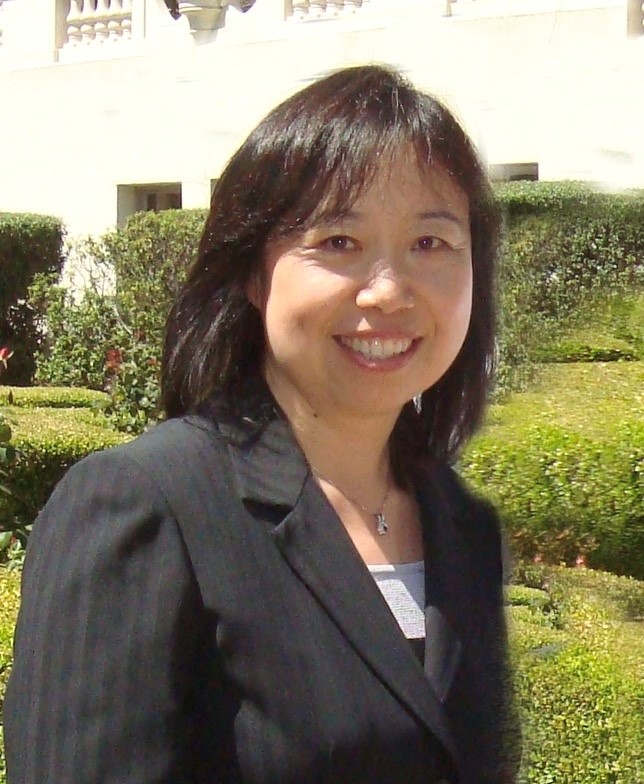
Quantifying the Impact of the Atmospheric Boundary Layer on Optical Signal Propagation
Recent developments of Free-space optical links for communication, directed energy beaming, EO/IR sensor imaging, and high-energy laser weapon systems utilize the optical/IR frequencies (electro-optical, or EO) of the electromagnetic wave spectra to propagate EO energy through the atmosphere. However, the atmosphere has non-negligible impacts on the EO beam through turbulence scintillation and attenuation by air molecules, aerosols, and fog. These effects are especially pronounced within the atmospheric boundary layer, which is the lowest hundreds of meters of the atmosphere. Along a given propagation path, turbulent refraction caused by temperature and water vapor fluctuations results in a defocused laser beam on the receiver/targets. Fog and aerosol particles and molecular constituents along the propagation path also absorb and/or scatter the incident laser beam, ultimately causing direct energy loss. These atmospheric effects from turbulence and fog/aerosols can be characterized through optical propagation measurements to obtain ‘path-integrated’ results or through environmental sampling of turbulence and aerosol absorption and scattering as input to optical propagation models.
In this seminar, I will introduce the atmospheric processes affecting EO propagation through the atmospheric boundary layer, focusing on atmospheric scintillation and attenuation by fog. Several field efforts will be presented to quantify the atmospheric impact on EO propagation, particularly those with concurrent measurements of optical scintillation/attenuation from the dynamic link measurements as well as from the in-situ aircraft measurements, allowing a direct comparison of the optical turbulence/aerosol properties and their effects for propagation. Results from these field efforts will also be presented to illustrate the complexity of the problem. I will also introduce our modeling efforts to quantify the characteristics of EO propagation in the atmosphere.
Dr. Qing Wang
Dr. Qing Wang is a Professor and the Associate Chair for Research in the Meteorology Department of the Naval Postgraduate School in Monterey CA. She also serves as an adjunct faculty member at Moss Landing Marine Laboratory in Moss Landing, CA. She obtained her B.S. degree and M.S. degree in Atmospheric Physics from Peking University in 1985 and 1988, respectively, and earned her Ph. D. Degree in Meteorology from the Pennsylvania State University in 1993. Before joining NPS in 1995, she was a postdoctoral fellow in the Advanced Study Program (ASP) at the National Center for Atmospheric Research (NCAR).
Dr. Wang is known for her contributions to the understanding of marine atmospheric boundary layer through aircraft and ship/buoy-based measurements and for using these observations to evaluate forecast and process-oriented models. She has been the lead PI for several multi-disciplinary and multi-institutional projects on quantifying the effects of the lower atmosphere on the propagation of radio waves and optical systems.
Dr. Wang has served on several subject area committees of the American Meteorological Society including Boundary Layer and Turbulence Committee, Coastal Processes Committee, and Air-Sea Interaction Committee. She is also a Commission F member of the U.S. National Committee – International Union for Radio Science (USNC-URSI). In 2019, she was invited to serve as a Navy representative on the Atmospheric Propagation Technical Area Working Group (TAWG). She is also a member of the US CLIVAR Working Group on “Mesoscale and Frontal-Scale Ocean-Atmosphere Interactions and Influence on Large-Scale Climate”. More recently, she has served as the UNOLS Fleet Improvement Committee (FIC) member to provide advice to assure the continuing excellence of the Academic Research Fleet (ARF) to serve the research community in the U.S.