By Noelle Lewis, MLML Geological Oceanography Lab
How and why we study temperature changes in the past 540 million years
You may have heard the phrase that looking to the past can help us understand the present and predict the future; but you probably didn’t think it would be necessary to go back in time over 500 million years. Global Average Temperatures are increasing rapidly due to human greenhouse gas emissions. To understand what a warmer future will be like, we can compare warming today to warming in the past.
Studying the Past
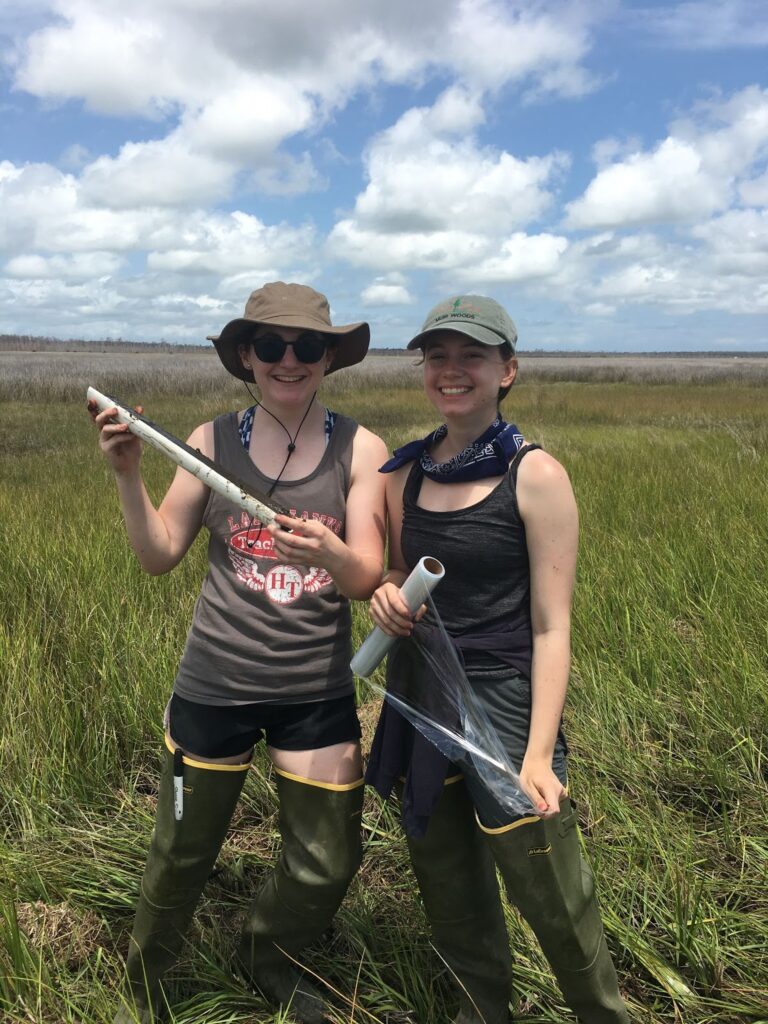
To measure what the temperature was hundreds of millions of years ago, you need to find the ruins of the past. If we were studying the Ancient Romans, this would be ruins like the Colosseum or the Roman Forum, but there weren’t buildings that far back in time; Instead we look at rocks or the sedimentary record.
The rock record can give us so much information about the past. This is one of the reasons I love studying geology. It is like being a detective. I can look at a rock in my hands and I can hypothesize the environment it formed in. It is like looking back in time.
Specific past temperatures can be determined by measuring certain properties (oxygen isotope ratios) in carbonate rocks and fossils. These measurements are useful when you want to measure a rapid increase or decrease in temperature. More gradual changes in temperature can be inferred from changes in the distribution of types of rocks - different rocks form in different environments. For example, coal forms in warm/wet environments and tillite forms in cold/dry environments from the movement of glaciers. Today increases in temperature are occurring at rates that are unprecedented compared to changes in temperature in Earth history. In the past, a rapid change in temperature may have occurred over thousands of years, unlike today when temperatures are occurring over hundreds of years.
Temperature Change without Humans
Humans are causing the climate change of today, but throughout most of Earth history, there weren’t any humans. Temperature changes from ice cold to very hot were normal and caused by natural phenomena. These cycles take place over timescales of 50 million to 100 million years. They are what geologists call icehouse (We are in an icehouse right now! It means that there is permanent ice on Earth’s North and South Poles) and hothouse (When there is no ice on the poles throughout the entire year) are caused by natural phenomena that take place over tens of millions of years to thousands of years.
- Icehouse - An icehouse is when there is permanent ice on the North and South Pole
- Hothouse - A hothouse is when there isn’t any ice on the poles throughout the whole year
Natural phenomena that cause long term changes in temperature (>50 million years) are caused by the movement of tectonic plates and changes in biological life like the evolution of plants. These events cause “slow and steady” changes in carbon dioxide (CO2) in the atmosphere because they all occur over very long time scales.
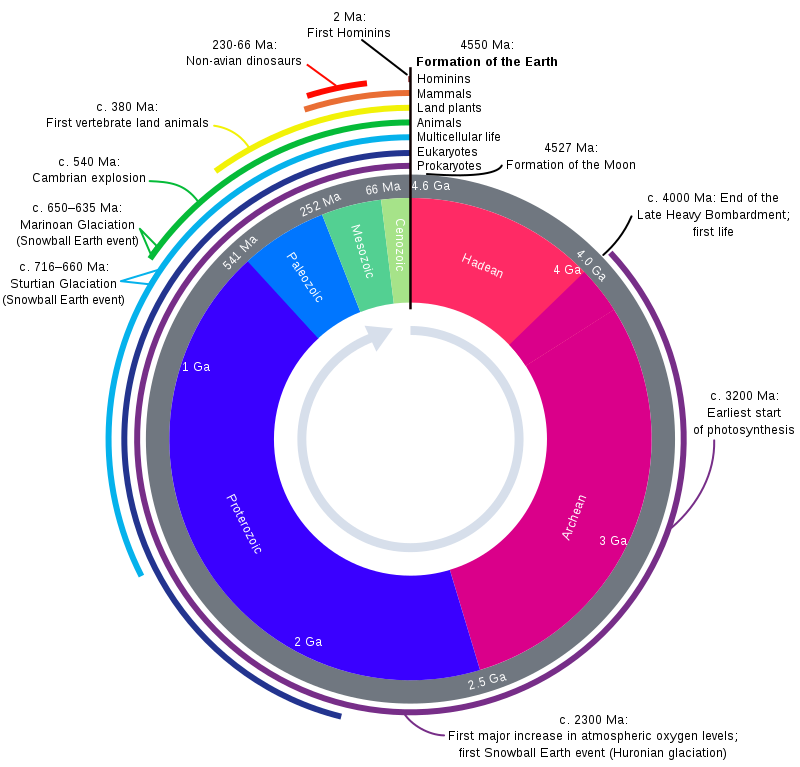
Rapid changes in temperature (< 1 million years) are caused by events like a Large Igneous Province (LIP) or a meteorite impact. LIPs are large volcanic eruptions that cause large amounts of CO2 to enter the atmosphere very quickly, which causes temperature to increase (2). When a meteorite hits Earth, the temperature temporarily decreases before going back to original temperatures. While both create rapid temperature changes by geological standards, LIPs occur over hundreds of thousands of years; whereas a meteorite impact can change temperatures over hundreds of years.
- Large Igneous Province (LIP) - Large Igneous Provinces are large volcanic eruptions that erupt in short periods of time (3)
On even shorter time scales (~20,000 years), changes in Earth’s orbit can alter temperature (these are called Milankovitch cycles). Milankovitch cycles are a combination of changes in how Earth orbits around the sun (eccentricity), how earth orbits around its own axis (precession), and the angle Earth’s axial tilt (obliquity).
Connecting Past to Present
On Earth today and in the past, climate can be split into five different groups, called the “Köppen Climate Belts.” Though the exact temperature and width of each belt changes over time, each belt has a distinct climate that corresponds to specific plants, animals, and geology. For example, the equator has a hot and humid climate with certain plants and animals that live there.
Because we know what plants, animals, and geology are found in specific climates, it is possible to make general interpretations about past climate through the rock record when those indicators are found.
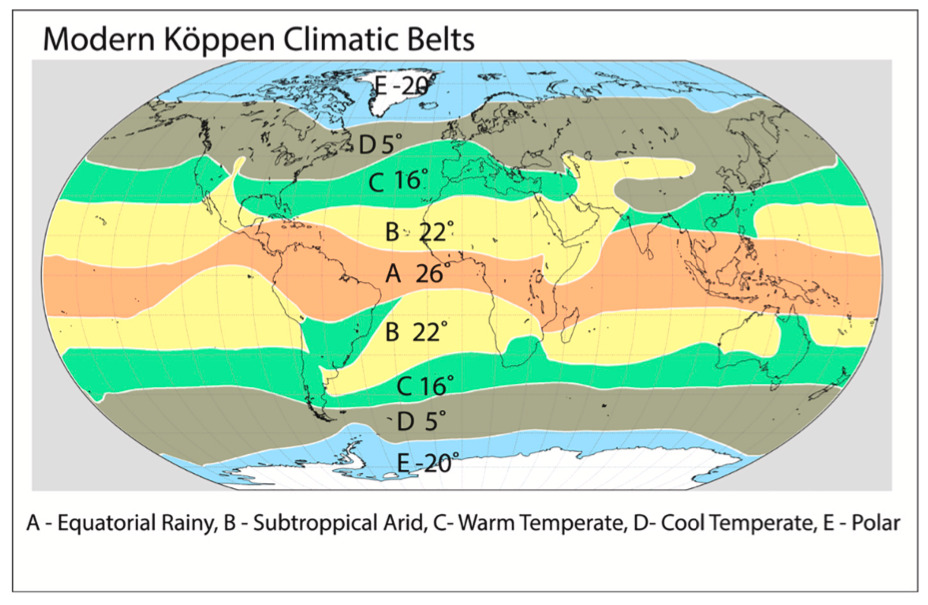
Temperature throughout the last 540 million years
Much of Earth’s history is not preserved in the rock record. However, starting about 540 million years ago there is a substantial record of past temperatures preserved in rocks (if you look at the pie chart above showing geologic time, Earth History began ~4.6 billion years ago, but there is very little rock record until the Paleozoic, which is the dark blue section of the pie). Temperature patterns in the last 540 million years can be split into groups of relatively warm and relatively cool periods. By describing how temperature has changed in the past, we can better understand how temperature will change in the future.
Cambro-Ordovician Hot House & Late Ordovician-Silurian Icehouse
540 Million Years Ago
During the Cambro-Ordovician hot house, temperatures were warm throughout the entire period. By the Middle Ordovician temperatures began to decrease,4 eventually entering the Late Ordovician-SIlurian Icehouse. Some explanations for the shift to cooler temperatures are an increase in weathering (5), the evolution of land plants (6), or a meteorite impact.
Siluro-Devonian Warm Period & Late Paleozoic Icehouse
426 Million Years Ago
Throughout the Siluro-Devonian Warm Period, temperatures fluctuated between warm and cool periods. Permanent ice did not form in the North and South Poles (Boucot et al. 2013).
Scientists infer temperature changes from evidence of changes in sea-level (7). Scientists also know that temperatures alternated between warm and cold throughout the period because the fossilized sea animals alternated between animals that live in warm and cold seawater.
365 Million Years Ago
The Late Paleozoic Icehouse spanned over 100 million years and there was permanent ice on the North and South poles. The Late Paleozoic Icehouse ended with the eruption of the West Siberian Large Igneous Province, which increased temperatures significantly (2).
Triassic Hothouse & Jurassic - early Cretaceous Cold Interval
253 Million Years Ago
High temperatures during the Triassic Hothouse were caused by large influxes of CO2 into the atmosphere due to eruptions of two Large Igneous Provinces (West Siberian Large Igneous Province and Central Atlantic Magmatic Province) (8). Evidence for the Triassic Hothouse includes fossils of dense forests at the poles (9). Both of these eruptions caused large extinction events because of how quickly CO2 enters the atmosphere; the amount of CO2 entering the atmosphere due to human activity today is occurring at a greater rate.
199 Million Years Ago
The Jurassic-early Cretaceous Cool Interval is made up of both cool and warm intervals. During this period, Earth’s climate was in between a hot house and icehouse. The mild conditions during this period are evidenced by rocks that form in cold conditions, along with temperate forest fossils in polar latitudes. The mixture of warm and cool period indicators indicates that temperatures were cold enough in winter for there to be ice but in summer temperatures were warm enough to support plant life.
Mid Cretaceous - Paleogene Hothouse & Late Cenozoic Icehouse
128 Million Years Ago
During the Mid Cretaceous - Paleogene Hothouse global average temperatures were greater than temperatures today. Temperatures gradually decreased until a massive meteorite impact in the Late Cretaceous (Permo-Triassic Impact). This is the impact that is hypothesized to have caused the Permo-Triassic Mass Extinction that killed the dinosaurs (75% of all species went extinct).
39 Million Years Ago
The Late Paleozoic Icehouse period is characterized by gradual cooling. Cooling during the Late Cenozoic Icehouse was caused by the formation of the Himalaya Mountains. After the mountains formed, weathering increased, which took CO2 out of the atmosphere and gradually decreased global temperatures.
Today
Before human activity changed the climate, we were in a glacial/interglacial cycle. Temperatures should be decreasing and ice sheets should be expanding in the North and South Poles. Instead, because of human created greenhouse gas emissions, global average temperatures are increasing and ice is melting.
There are a lot of unknowns about what the long term consequences of climate change will be. Describing how temperatures have changed in the last 540 million years can be very useful for this. It can help us understand what causes climate to change and what happens when climate changes. It will help us be better prepared for the obstacles we will face in the future. Scotese et al. 2021, (the article that informed the research discussed in this blog post) acknowledges that this paleotemperature timeline is not complete and will require further research but they argue that this is just the first step in understanding how past temperatures have changed over Earth history and integral to our understanding of our current climate crisis. Thinking about climate change can be very scary, but studying the past gives me hope that we will be able to make a plan and create a better future.
Sources
1. Scotese, C., Song, H., Mills, B.J.W., Meer, DG. (2021). Phanerozoic Paleotemperatures: The Earth’s Changing Climate during the Last 540 million years. Earth-Science Reviews. 215. 10.1016/j.earscirev.2021.103503.
2. Ernst, R.E., Youbi, N., (2017). How Large Igneous Provinces affect global climate, sometimes cause mass extinctions, and represent natural markers in the geological record. Palaeogeography, Palaeoclimatology, Palaeoecology 478, 30–52. https:// doi.org/10.1016/j.palaeo.2017.03.014.
3. Coffin, M. F., and Eldholm, O., (1994). Large igneous provinces: Crustal structure, dimensions, and external consequences, Rev. Geophys., 32( 1), 1– 36, doi:10.1029/93RG02508.
4. Trotter, J.A., Williams, I.S., Barnes, C.R., L ́ecuyer, C., Nicoll, R.S., (2008). Did Cooling Oceans Trigger Ordovician Biodiversification? Evidence from Conodont Thermometry. Science 321 (5888), 550–554. https://doi.org/10.1126/ science.1155814.
5. Swanson-Hysell, N.L., Macdonald, F.A., (2017). Tropical weathering of the Taconic Orogeny as a driver for Ordovician cooling. Geology, v 45, 719–722. https://doi. org/10.1130/G38985.1.
6. Lenton, T.M., Crouch, M., Johnson, M., Pires, N., Dolan, L., 2012. First plants cooled the Ordovician. Nature Geoscience 5, 86–89.
7. Stock, C.W., (2005). Devonian stromatoporoid originations, extinctions, and paleobiogeography: how they relate to the Frasnian-Famennian extinction. In: Understanding Late Devonian and Permian-Triassic Biotic and Climatic Events; Towards an Integrated Approach, D.J. Over, J.R. Morrow, and P.B. Wignall (editors), Developments in Palaeontology & Stratigraphy, v. 20. Elsevier, Amsterdam, pp. 71–92.
8. Ernst, R.E., (2014). Large Igneous Provinces. Cambridge University Press, Cambridge, UK, 653 pp.
9. Ziegler, A.M., Parrish, J.M., Jiping, Y., Gyllenhaal, E.D., Rowley, D.B., Parrish, J.T., Sahngyou, N., Behher, A., Hulver, M.L., (1994). Early Mesozoic phytogeography and climate. In: Allen, J., Hoskins, B.J., Sellwood, B.W., Spicer, R.S., Valdes, P.J. (Eds.), Paleoclimates and Their Modelling: with Special Reference to the Mesozoic Era. Chapman and Hall, London, pp. 89–97.